- Understanding Caltech's Motion Analysis Theories
- The Mathematics Behind Motion: A Brief Overview
- Real-world Applications: Bridging Theory and Practice
- Case Study: Enhancing Robotic Arm Efficiency
- Integrating Motion Analysis into Engineering Assignments
- Step 1: Define the Problem Statement
- Step 2: Choose the Appropriate Motion Model
- Step 3: Mathematical Formulation and Analysis
- Step 4: Validation through Simulation
- Future Trends and Innovations in Motion Analysis
- AI-Driven Motion Analysis: A Glimpse into Tomorrow
- Conclusion:
Motion analysis stands as a cornerstone in the realm of engineering, offering a profound understanding of the intricate dynamics governing mechanical systems. Caltech, celebrated for its substantial contributions to the fields of science and engineering, provides a theoretical framework that leaves an indelible mark on the landscape of motion analysis. This blog embarks on a journey to explore the practical implications of applying Caltech's motion analysis theories in the context of engineering assignments, peeling back the layers to reveal nuanced insights and effective problem-solving strategies.
Caltech's theories, rooted in mathematical precision and practical applicability, serve as a guiding light for engineers grappling with the complexities of motion within mechanical systems. Whether it's understanding the velocity vectors of a robotic arm or analyzing the acceleration patterns of a moving vehicle, the theories from Caltech lay a robust foundation for comprehending and manipulating these dynamic behaviors.
As engineers, our pursuit is not merely theoretical; it extends into the tangible realm of problem-solving. Caltech's motion analysis theories provide the lens through which we can dissect and address real-world challenges. This practicality is what sets these theories apart, turning abstract mathematical concepts into powerful tools for optimizing the performance of a diverse array of structures and mechanisms.
In the realm of engineering assignments, the application of Caltech's motion analysis theories becomes a strategic approach to unraveling complex problems. Picture a scenario where a team of engineers is tasked with designing a conveyor system for an automated assembly line. Caltech's theories come into play as they analyze the motion patterns of various components, optimizing the conveyor's speed and trajectory to ensure seamless and efficient production processes.
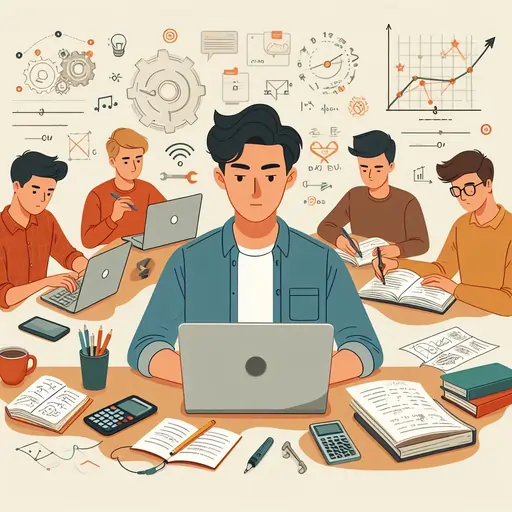
The synergy between theory and practice becomes palpable in case studies where Caltech's motion analysis theories are the linchpin for successful problem resolution. Consider a project where engineers are tasked with improving the precision of a CNC machine. Through a meticulous application of Caltech's theories, they identify and mitigate sources of vibration, ultimately enhancing the machine's accuracy and performance.
In essence, the integration of Caltech's motion analysis theories into engineering assignments is akin to wielding a sophisticated toolkit. Each theory is a specialized instrument, designed to address specific aspects of motion dynamics. Engineers navigate through the toolbox, selecting the right theories for the job at hand, be it analyzing the harmonic motion of a pendulum or predicting the trajectory of a projectile.
The seamless integration of motion analysis theories into engineering assignments follows a logical sequence. Engineers start by defining the problem statement, outlining the specific challenges they aim to address. Once the problem is crystallized, the next step involves choosing the most appropriate motion model from Caltech's arsenal of theories.
The heart of the process lies in the mathematical formulation and analysis, which is crucial to solving your Motion Analysis assignment. Engineers translate the real-world intricacies of motion into equations, applying Caltech's theories to derive meaningful insights. This mathematical journey is not a mere exercise in numbers; it's a pathway to understanding the underlying principles governing motion in a given engineering context.
Validation through simulation adds another layer of assurance to the proposed solutions. Engineers leverage advanced simulation tools to test their hypotheses, ensuring that the theoretical improvements align with the practical realities of the system. This iterative process of analysis, formulation, and validation is the essence of applying Caltech's motion analysis theories in engineering assignments.
Looking ahead, the future of motion analysis in engineering holds exciting prospects. As technology evolves, so does the toolkit available to engineers. Artificial intelligence emerges as a formidable ally, promising to augment the capabilities of motion analysis by introducing adaptive algorithms and predictive modeling. The intersection of AI and Caltech's motion analysis theories opens a gateway to a new era of precision and efficiency in engineering solutions.
Understanding Caltech's Motion Analysis Theories
Caltech's distinctive approach to motion analysis seamlessly integrates mathematical precision with real-world applicability. At its core, the foundation of Caltech's motion analysis theories rests upon the mastery of fundamental concepts such as velocity and acceleration, and an intricate understanding of how these factors dynamically interact within mechanical systems. Engineers, by delving into these theories, acquire a robust toolkit that empowers them to dissect, interpret, and enhance the performance of a diverse range of structures and mechanisms.
Velocity, a fundamental parameter in motion analysis, encapsulates the rate at which an object changes its position concerning time. Caltech's theories guide engineers in dissecting the nuances of velocity, enabling them to discern the intricacies of motion within a given system. Acceleration, the derivative of velocity with respect to time, further enriches the analysis by shedding light on the changes in velocity. Engineers armed with this knowledge can unravel the underlying factors influencing the acceleration of a system, leading to more informed decisions in engineering assignments.
The interplay between velocity and acceleration within a mechanical system forms the bedrock of Caltech's motion analysis theories. This symbiotic relationship allows engineers to explore the dynamic behavior of objects in motion, paving the way for precise calculations and strategic optimizations. As engineers navigate the complexities of motion in the realm of Caltech's theories, they not only decode the language of mechanical systems but also gain a profound appreciation for the elegance with which mathematics converges with real-world engineering challenges.
The Mathematics Behind Motion: A Brief Overview
To embark on the journey of applying Caltech's motion analysis theories, a solid grasp of the underlying mathematics is essential. This section breaks down the key mathematical principles that form the basis of motion analysis in engineering assignments. From calculus to vector algebra, we explore the tools necessary to navigate the complexities of motion in the engineering realm.
In the realm of motion analysis, calculus plays a pivotal role. The concept of derivatives allows engineers to precisely define and analyze rates of change in a system, essential for understanding dynamic processes. Integrals, on the other hand, facilitate the accumulation of infinitesimally small changes, providing insights into quantities such as displacement and velocity over time.
Vector algebra emerges as a powerful tool in motion analysis, enabling the representation of quantities with both magnitude and direction. Engineers leverage vectors to describe motion in three-dimensional space, crucial for scenarios where movement occurs along multiple axes. Understanding vector operations and their application to displacement, velocity, and acceleration vectors is fundamental in unraveling the intricacies of motion.
Matrix algebra, another mathematical cornerstone, finds its application in system dynamics. Engineers utilize matrices to model interconnected mechanical components, allowing for the simultaneous analysis of multiple variables. This proves invaluable when studying complex systems where various factors influence motion simultaneously.
Furthermore, differential equations arise naturally in motion analysis, offering a mathematical framework to express how quantities change concerning each other. Engineers employ differential equations to model dynamic systems, enabling predictions of future behavior based on the system's current state and governing principles.
Real-world Applications: Bridging Theory and Practice
Motion analysis transcends theoretical frameworks, finding tangible relevance in the everyday challenges engineers encounter. In the realm of machinery design, Caltech's motion analysis theories have emerged as indispensable tools for achieving efficiency and precision. Engineers tasked with creating cutting-edge machinery delve into the intricacies of motion analysis to optimize the performance of gears, cams, and other mechanical components. By applying Caltech's theories, they can fine-tune the motion profiles, minimizing energy losses and ensuring seamless operation.
Robotic movements, a cornerstone of modern automation, present another arena where Caltech's motion analysis theories shine. Engineers grapple with the intricacies of robotic kinematics and dynamics, striving to enhance agility, accuracy, and speed. Caltech's contributions guide them in developing algorithms and control strategies that unlock the full potential of robotic systems. From assembly lines to surgical robots, the marriage of theory and practice in motion analysis revolutionizes how robots interact with and respond to their environments.
Moreover, in the aerospace industry, where precision is paramount, Caltech's motion analysis theories play a pivotal role. Engineers engaged in the design and optimization of aircraft components harness these theories to predict and control the motion of aircraft during flight. Understanding the aerodynamics and fluid dynamics involved allows for the creation of aircraft with improved stability, fuel efficiency, and overall performance.
Case Study: Enhancing Robotic Arm Efficiency
In this detailed case study, we delve into the practical application of Caltech's motion analysis theories, specifically examining their transformative impact on a robotic arm within an industrial setting. Engineers, armed with the theoretical foundations, strategically optimized the arm's trajectory by meticulously factoring in crucial elements such as inertia and friction. This thoughtful analysis and subsequent adjustments didn't just enhance the efficiency of the robotic arm; it led to a remarkable surge in productivity, minimizing downtime to a significant extent. The success of this case study underscores the tangible benefits that arise from a judicious integration of Caltech's motion analysis theories into real-world engineering challenges.
Integrating Motion Analysis into Engineering Assignments
Now that we've explored the theoretical and practical aspects of Caltech's motion analysis theories, it's time to discuss how engineers can seamlessly integrate these concepts into their assignments. This section provides a step-by-step guide, offering tips and tricks to tackle engineering problems through the lens of motion analysis.
Step 1: Define the Problem Statement
Effective motion analysis begins with a clear understanding of the engineering problem at hand, a critical phase that warrants further exploration. Whether it's designing a groundbreaking mechanism or troubleshooting an existing system, the process of defining the problem statement involves a comprehensive analysis of the system's intricacies. Engineers must delve into the specifics, identifying key challenges and constraints that shape the trajectory of the project. This step sets the stage for a targeted application of motion analysis theories, ensuring that the subsequent steps are aligned with the unique demands posed by the engineering problem.
Step 2: Choose the Appropriate Motion Model
Caltech's rich array of motion theories offers a diverse set of tools, each tailored to specific scenarios. Engineers, in the pursuit of precision and relevance, must navigate this theoretical landscape adeptly. Careful consideration is required to select the most fitting motion model for the assignment at hand. Factors such as the nature of motion—be it linear or rotational—and the complexity of the mechanical system must be weighed. This section delves deeper into the nuanced decision-making process, providing insights into the strategic choices that underpin successful motion analysis in engineering assignments.
Step 3: Mathematical Formulation and Analysis
With the problem statement clarified and the motion model selected, the engineering journey advances into the realm of mathematical abstraction. This stage involves a transformative process where the real-world scenario is translated into a mathematical framework. Engineers are guided through the intricacies of formulating equations that encapsulate the essence of motion within the system. The application of relevant motion analysis theories takes center stage, as engineers conduct a meticulous mathematical analysis. This step, crucial for precision and accuracy, bridges the conceptual and practical realms, laying a robust foundation for subsequent phases of the assignment.
Step 4: Validation through Simulation
Before any alterations are made to a physical system, validation becomes paramount. Engineers turn to simulation tools, an indispensable ally in the validation process. This step explores the symbiotic relationship between simulation software and Caltech's motion analysis theories. The aim is to ensure that proposed solutions align seamlessly with real-world expectations. From refining robotic movements to optimizing the performance of complex machinery, this section sheds light on the iterative nature of motion analysis, where simulation serves as a virtual proving ground for engineering innovations.
Future Trends and Innovations in Motion Analysis
As technology continues its relentless advance, the field of motion analysis in engineering stands on the precipice of transformative change. The future promises a dynamic interplay of innovation and practicality, with emerging trends poised to reshape how engineers navigate the intricate realm of motion analysis. Artificial intelligence (AI) will take center stage, driving simulations with unprecedented accuracy and efficiency. Engineers can anticipate harnessing the power of AI algorithms to unravel complex motion dynamics, facilitating quicker and more precise problem-solving.
Moreover, the integration of virtual reality (VR) into motion analysis processes holds immense potential. Engineers may find themselves immersed in virtual environments, allowing for immersive exploration and analysis of complex mechanical systems. This convergence of AI-driven simulations and virtual reality interfaces is set to redefine the boundaries of motion analysis, unlocking new dimensions of understanding and insight for the engineering community. As these exciting possibilities unfold, the landscape of motion analysis will undoubtedly undergo a paradigm shift, ushering in an era where technology amplifies the capabilities of engineers in unprecedented ways.
AI-Driven Motion Analysis: A Glimpse into Tomorrow
As technology advances, the integration of artificial intelligence (AI) into engineering practices has become more pronounced, revolutionizing traditional approaches to problem-solving. Motion analysis, a critical aspect of engineering, is undergoing a transformative shift through the incorporation of AI algorithms. These algorithms, equipped with machine learning capabilities, have the potential to elevate the accuracy and efficiency of motion analysis to unprecedented levels.
AI-driven motion analysis operates on the principle of continuous learning and adaptation. These algorithms can analyze vast datasets, identifying patterns and correlations that may elude traditional analytical methods. This not only enhances the precision of motion predictions but also enables the identification of subtle nuances in complex mechanical systems.
Moreover, AI algorithms bring a level of adaptability to motion analysis that is unparalleled. As engineering scenarios evolve, these algorithms can dynamically adjust their parameters, ensuring that the analysis remains relevant and effective. This adaptability proves invaluable in scenarios where real-world conditions may deviate from initial expectations.
The marriage of AI and motion analysis also opens new avenues for innovation. Engineers can explore unconventional solutions and design paradigms, confident in the AI's ability to analyze and optimize these novel approaches. The iterative nature of AI-driven analysis facilitates rapid prototyping and experimentation, fostering a culture of continuous improvement in engineering practices.
Conclusion:
In conclusion, Caltech's motion analysis theories emerge as a cornerstone, laying a robust foundation for engineers as they delve into the intricate realms of understanding and optimizing the dynamic behavior inherent in mechanical systems. The symbiotic relationship between theoretical knowledge and practical application forms the bedrock upon which engineers navigate the complexities of their assignments.
As engineers immerse themselves in the theoretical tenets put forth by Caltech, they gain a nuanced understanding of motion dynamics that goes beyond textbook principles. This deep comprehension equips them to unravel the complexities inherent in real-world engineering challenges, enabling a holistic approach to problem-solving.
The significance of this synthesis between theory and practice becomes palpable when engineers confront complex assignments. Armed with the knowledge distilled from Caltech's theories, they confidently traverse the terrain of intricate mechanical systems, addressing challenges with a precision born out of a comprehensive understanding of motion principles.
Moreover, the practical application of Caltech's motion analysis theories instills a sense of pragmatism in engineers. It compels them to consider not just the theoretical ideals but also the pragmatic constraints and nuances of the physical world. This real-world perspective enhances the efficacy of engineering solutions, ensuring they are not only theoretically sound but also practically viable and sustainable.
The empowerment derived from Caltech's motion analysis theories extends beyond the immediate confines of assignments. It becomes a catalyst for broader advancements in the field of engineering. Engineers, armed with a solid grasp of motion dynamics, contribute to the evolution of technologies, the optimization of existing systems, and the conceptualization of innovative solutions that push the boundaries of what is possible.
This empowerment, however, is not static but dynamic, mirroring the very essence of the systems engineers seek to understand. As technology evolves, as new challenges emerge, and as engineering landscapes transform, the principles derived from Caltech's motion analysis theories remain a guiding light. They serve as a compass, guiding engineers through uncharted territories and inspiring the development of novel methodologies and approaches.