- 1. Basics of Control Systems
- Definition and Purpose
- Components of a Control System
- Types of Control Systems
- 2.Control System Modeling
- Mathematical Modeling
- Block Diagram Representation
- 3. Control System Analysis
- Stability Analysis
- Time-Domain Analysis
- Frequency-Domain Analysis
- 4. Control System Design
- Proportional-Integral-Derivative (PID) Control
- State-Space Control
- Conclusion
Modern electrical engineering relies heavily on control systems because they give us the ability to precisely and effectively regulate and manage dynamic systems. Electrical engineering students must understand the fundamental principles of control systems in order to automate processes in industrial settings, regulate the temperature in smart homes, or stabilise the flight of aircraft. This blog aims to offer a thorough overview of control systems with a focus on the core ideas that underpin this discipline. We will examine how control systems maintain stability, accuracy, and dependability in the face of disturbances and uncertainties by delving into the fundamentals of control systems, including their definition, purpose, and components. We will also examine the various kinds of control systems, including closed-loop and open-loop systems, and highlight each one's benefits and drawbacks. In addition, we'll talk about modelling control systems, stability analysis methods, time-domain and frequency-domain behaviour, and well-liked control systems design methods like PID control and state-space control. Electrical engineering students will be well-prepared to take on assignments and projects related to control systems with assurance and expertise if they are armed with this knowledge.
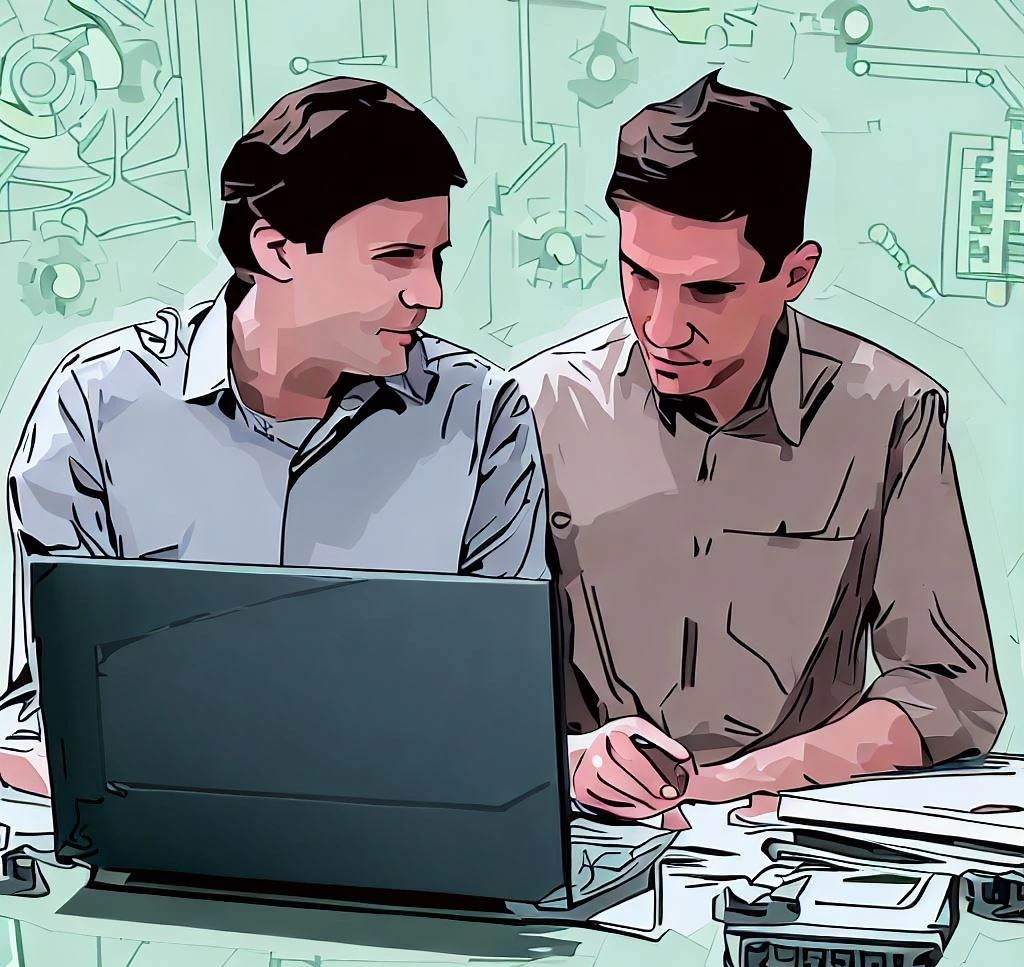
1. Basics of Control Systems
Electrical engineering is built on control systems, which enable precise monitoring and regulation of dynamic processes. Control systems are fundamentally made to direct and manage the actions of various systems, including mechanical, electrical, chemical, and even biological ones. The main goal of control systems is to maintain stability, accuracy, and dependability even in the presence of disturbances and uncertainties in order to achieve desired outputs or performance. Control systems are made up of a number of essential parts to achieve this. In the beginning, sensors are used to measure the system's output or other pertinent variables and give the controller feedback. The controller analyses this feedback and produces the proper control signals to modify the behaviour of the system. Actuators receive these control signals and translate them into actual system actions or adjustments. The output of the system is lastly continuously monitored and compared to the desired output using a feedback loop. Control systems can correct errors and improve performance, stability, and robustness by incorporating feedback. Understanding these fundamental elements and ideas is necessary for fully appreciating the complexities of control systems and for completing assignments and projects in the field of electrical engineering.
Definition and Purpose
Control systems are created to direct, command, and regulate the actions of dynamic systems in order to produce the results or performance that are desired. The nature of these systems can be mechanical, electrical, chemical, or even biological. Maintaining stability, accuracy, and dependability in the face of disturbances, uncertainties, and variations is the main goal of a control system.
Components of a Control System
There are typically four main parts to a control system:
- Sensors:Sensors take measurements of the system's output or pertinent variables and give the controller feedback.
- Controller: The controller analyses sensor feedback and produces the necessary control signals to modify the behaviour of the system.
- Actuators:These devices take control signals and translate them into system adjustments or physical actions.
- Feedback Loop: Based on the discrepancy between the desired output and the actual output, the feedback loop enables continuous monitoring and adjustment.
Types of Control Systems
Two general categories can be used to classify control systems:
- Open-loop Control Systems: In open-loop systems, the control action is chosen solely based on the command input, taking the system's output into no account. These systems are vulnerable to disturbances and uncertainties because they lack feedback.
- Closed-loop Control Systems: Closed-loop controls, also referred to as feedback controls, use feedback to compare the system's output to the desired output on a continuous basis. This feedback allows for error correction and enhances the functionality, stability, and robustness of the system.
2.Control System Modeling
Understanding and analyzing the behavior of control systems begins with control system modelling. The dynamics of a control system are expressed mathematically using equations and transfer functions. Engineers can gain insights into the behavior and performance of the system by using these models, which capture the relationship between the system's input and output signals. In particular, transfer functions show how a system reacts to various input signals in the frequency domain. Engineers are able to evaluate the stability, frequency response, and other properties of the control system by looking at and analyzing the transfer function. Block diagrams are frequently used to visually represent control systems in addition to mathematical modelling. Block diagrams demonstrate the signal flow and interactions between various elements by representing the various control system components as interconnected blocks. By streamlining the analysis and design process, this graphical representation makes it simpler to comprehend the system's architecture and spot potential problems or improvements. Engineers can use control system modelling as a potent tool to simulate, analyze, and optimize control systems, which will ultimately improve engineering applications' performance, stability, and efficiency.
Mathematical Modeling
Understanding the behavior and characteristics of control systems requires mathematical modelling. It involves using mathematical equations and transfer functions to express the dynamics of the system. The relationship between the system's input and output signals is represented by transfer functions in the frequency domain.
Block Diagram Representation
Block diagrams are frequently used to graphically represent control systems. They are made up of different building blocks that stand in for the different system parts and how they are connected. Block diagrams make it simpler to analyze and design complex systems by giving a visual representation of the control system's structure.
3. Control System Analysis
An essential step in assessing the behavior and effectiveness of control systems is control system analysis. To evaluate the system's stability, transient response, and frequency response, a variety of techniques and methods are applied. A crucial component of control system analysis is stability analysis, which predicts whether the system will remain stable or experience oscillations and instability. Stability can be evaluated using methods like the Routh-Hurwitz stability criterion, Nyquist stability criterion, and Bode stability criterion. The goal of the time-domain analysis is to examine how the system reacts to various input signals over time while taking into account variables like rise time, settling time, peak time, and overshoot. This analysis sheds light on the operation and momentary behaviour of the system. On the other hand, frequency-domain analysis looks at the system's behaviour in the frequency range. Analysis of the system's frequency response, gain margin, phase margin, and stability margins is done using methods like Bode plots and Nyquist plots. Engineers can improve the performance, stability, and robustness of the system by conducting control system analysis to better understand the system's characteristics, spot potential problems or limitations, and make knowledgeable design decisions.
Stability Analysis
A crucial component of the design of a control system is stability analysis. It establishes whether the system can maintain stability and prevent oscillations or instability. Stability can be evaluated using methods like the Routh-Hurwitz stability criterion, Nyquist stability criterion, and Bode stability criterion.
Time-Domain Analysis
Studying a system's response to various input signals over time is called time-domain analysis. In order to assess the system's performance and transient behavior, it concentrates on variables such as rise time, settling time, peak time, and overshoot.
Frequency-Domain Analysis
The frequency domain behavior of a system can be examined using frequency-domain analysis. It offers information about the system's gain margin, phase margin, and stability margins. Frequency-domain analysis uses methods like Nyquist plots and Bode plots.
4. Control System Design
Control system design is a crucial stage in the development and implementation of effective control systems. It involves the application of engineering principles and techniques to create control strategies that achieve desired system performance and stability. One commonly used control design strategy is the Proportional-Integral-Derivative (PID) control. PID control combines proportional, integral, and derivative actions to achieve optimal control. The proportional term provides immediate response based on the error between the desired and actual outputs, the integral term eliminates steady-state error by integrating the error over time, and the derivative term improves system stability by anticipating future trends based on the rate of change of the error. Another approach to control system design is state-space control, which represents the system's dynamics using a set of first-order differential equations. This method enables advanced control techniques such as pole placement, optimal control, and robust control. Control system design aims to optimize system performance, stability, and robustness by selecting appropriate control strategies, tuning control parameters, and implementing effective control algorithms. Through careful design, engineers can achieve precise and efficient control of dynamic systems, contributing to advancements in various industries and applications.
Proportional-Integral-Derivative (PID) Control
One of the most popular control methods is PID control. To achieve the desired system performance, it combines proportional, integral, and derivative actions. The integral term eliminates steady-state error, the derivative term increases system stability, and the proportional term offers immediate response.
State-Space Control
The dynamics of the system are represented by a set of first-order differential equations in state-space control. This strategy permits more sophisticated control design methods like optimal control, robust control, and pole placement.
Conclusion
In conclusion, control systems are essential to electrical engineering because they allow for precise monitoring and regulation of dynamic processes. Students can establish a strong foundation for tackling assignments and projects in this field by understanding the fundamentals of control systems, including their components and types. By using transfer functions and block diagrams, control system modelling enables engineers to mathematically represent the system's dynamics and examine its behaviour. Techniques for analysing control systems that focus on system performance, stability, and response characteristics include stability analysis, time-domain analysis, and frequency-domain analysis. Furthermore, effective methods for achieving desired system performance and stability are provided by control system design strategies like PID control and state-space control. Electrical engineering students can approach control system assignments and projects with assurance and competence by mastering these fundamental ideas and methods. Engineers can advance automation, robotics, industrial processes, and many other areas where precise control is essential by modelling, analysing, and designing control systems. Electrical engineering's fascinating and fundamental control systems are one area in which success depends on having a firm grasp of the underlying ideas.